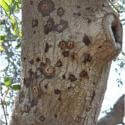
Polyphagous shot hole borer Euwallacea fornicatus s.s. and Kuroshio shot hole borer Euwallacea kuroshio are vectors of “Fusarium dieback” disease caused by Fusarium euwallacea and Fusarium kuroshium.
NOTE: the Polyphagous and Kuroshio shot hole borers and their associated fungi – primarily Fusarium – attacking avocados and other trees in California are completely unrelated organisms to Laurel wilt, despite both being beetle-fungi complexes that effect avocado. Click here to read about Laurel wilt, an ambrosia beetle Xyleborus glabratus and associated fungus Harringtonia lauricola.
Ambrosia beetles are prominent among introduced species. Their invasions are facilitated by their cryptic habits and ecologies, wide host ranges, and specialized breeding systems which allow extremely low populations to start an infestation. This breeding system often results in low genetic diversity in introduced ranges, but this has not hampered their success (Bierman et al. 2022). Ambrosia beetles serve as a vector for various fungi which nourish the beetles’ larvae. Most of these fungi don’t harm living trees, but some do.
Life Cycle of Ambrosia beetles
Ambrosia beetles develop through four life stages: egg, larva, pupa, and adult. Adult females bore through tree bark, create galleries (tunnels), and introduce the fungi (which they carry in specialized organs near their mouths). The female lays eggs in the galleries where the fungus grows. When the eggs hatch, the larvae eat the fungus. Larvae develop through four increasingly larger instars. After pupating, the new adult females mate with the males while in the galleries. Then pregnant females carrying the fungus exit the tree through the entry holes created by their mothers and re-infest the same tree or fly to seek new hosts (UC IPM). An unmated female can also start a new infestation through parthenogenesis. If the female lays unfertilized eggs they will become males, and once the larvae mature, the previously unmated female can mate with her male prodigy and subsequently produce female offspring (from fertilized eggs) thus perpetuating the colony (Normark et al. 1999).
California has been invaded by three ambrosia beetle species in the Euwallacea genus, Euwallacea fornicatus s.s., Euwallacea kuroshio, and Euwallacea interjectus. The beetles vector three previously undescribed species of fungus that cause disease in host trees: Fusarium euwallacea, Fusarium kuroshium, and Fusarium floridanum.
These three species look almost identical to one another, and to two further species, Euwallacea perbrevis and Euwallacea fornicatior; the only reliable way to tell them apart is by looking at their DNA (Gomez et al. 2018, Smith et al. 2019). Collectively, these species have been termed the Euwallacea fornicatus species complex. Some of the species, especially E. fornicatus s.s., appear in more than one haplotype, that is, genetic variant). Various members of the Euwallacea fornicatus species complex have invaded countries around the world:
- Euwallacea fornicatus s.s. has successfully established in the United States (California), Israel, South Africa, and Australia. In the U.S. it was first detected in southern California in 2003 (Rabaglia, 2006), and in Hawaii in 2007 (Mitchell and Maddox 2010; Rugman-Jones et al. 2020). In Hawaii, it is confirmed from Oahu and the Big Island (Rugman-Jones et al. 2020). The remaining islands have not been extensively surveyed, but undetermined members of the species complex have been recorded from Maui, Kaua`i and Moloka`i (Rugman-Jones et al. 2020). E. fornicatus s.s. was first recorded in Israel in 2009 and in South Africa in 2012. More recently, the species has successfully invaded Western Australia. In addition, since 2017 E. fornicatus s.s. has been detected and eradicated from several greenhouses across Europe. A common feature of the invasive populations in California, Israel, and South Africa is that they all share a particular haplotype, H33. This suggests that these three invasive populations, on three separate continents, might share a common source – possibly Vietnam.
- Euwallacea kuroshio has successfully established in the U.S., from which it has spread to Mexico. It was first detected in parts of San Diego County, California, in late 2013 and was subsequently confirmed across the border in Tijuana, Mexico, in 2015 (Garcia-Avila et al. 2016). This species has not been detected anywhere else outside of its native range.
- Euwallacea interjectus and its associated fungus Fusarium floridanum was invasion was found in Santa Cruz County in October 2024. Like several other non-native shothole borers in the same genus already known to be in California, Euwallacea interjectus is native to Southeast Asia. https://www.santacruzcountyca.gov/portals/0/county/CAO/press%20releases/2024/BorerUpdate.12112024.pdf So far, the newly detected infestation has affected primarily box elders (Acer negundo). Other tree species — California sycamore (Platanus racemose), coast live oak (Quercus agrifolia), arroyo willow (Salix lasiolepis), red willow (Salix laevigata), & black cottonwood (Populus balsamifera ssp. trichocarpa) — have also been attacked. The infestation extends across at least 75 acres (CDFA proposal). While it is too early to know precisely, E. interjectus is expected to pose a risk to tree urban, wildland and agricultural landscapes similar to that already manifested by Euwallacea fornicatus s.s., and Euwallacea kuroshio (KSHB). This infestation is outside the high-risk areas identified by a new model developed by Lynch et al. (2025).
- A fourth member of the species complex, E. perbrevis, has successfully established in the U.S. It is thought to have been introduced into Hawaii over a century ago and has been confirmed from the islands of Maui, Oahu, and the Big Island (Stouthamer et al. 2017; Rugman-Jones et al. 2020). The remaining islands have not been extensively surveyed, but undetermined members of the species complex have been recorded from Kaua`i and Moloka`i (Rugman-Jones et al. 2020). E. perbrevis has also established in Florida after initial detection in 2002 (Rabaglia, 2006). E. perbrevis has also been long-established in northern Queensland, Australia; this region might be part of its native range. Additionally, E. perbrevis was recently detected in nurseries in the Netherlands, but has since been reported as eradicated.
Genetic variation within E. fornicatus s.s. and E. kuroshio has been used to infer possible origins of the different invasive populations. However, it should be noted that populations in large parts of the native range of these species are severely under-sampled (e.g., India, Sri Lanka, Bangladesh, Myanmar, Cambodia, and Laos); even in regions with sufficient sampling, it is highly likely that the full range of genetic variation has not been recorded (e.g., in Vietnam and Thailand). As such, any inferences on the origin of invasive populations should be interpreted cautiously.
That said, to date, approximately 43 haplotypes (genetic variants) of E. fornicatus s.s. have been identified around the world, with the greatest diversity (number of haplotypes) found in the native areas of Taiwan (16 haplotypes), Vietnam (13 haplotypes), and China (8 haplotypes) (P. Rugman-Jones, pers. comm). The most widespread/dominant invasive haplotype is Haplotype 33 (H33) which, as noted previously, has been introduced to Israel, California, and South Africa. In the native range, H33 has only been detected in Vietnam, around Hanoi and Ho Chi Minh City (Stouthamer et al. 2017), suggesting a common origin of these invasive populations. This raises several questions. Has this haplotype’s ability to spread to distant locations been facilitated by its native range being close to major ports in Vietnam? Or have earlier introductions provided opportunities to spread to other regions, that is, the “bridgehead effect”? Or is H33 genetically superior in some way to other haplotypes, favoring its establishment? (Bierman et al. 2022).
In addition to H33, the introductions in California and South Africa involved further haplotypes that are unique to those receiving regions and may indicate alternative sources and/or multiple introduction events. In California H33 is accompanied by H35 (Stouthamer et al. 2017), and in South Africa, H38 is also found (Bierman et al. 2022). H35 has not been detected anywhere other than California, but H38 is known to occur in native areas of Vietnam and Taiwan. The introduction in Hawaii is of a single haplotype that has not yet been detected elsewhere, and the introduction in Europe (subsequently eradicated) also involved several haplotypes not detected elsewhere (Bierman et al. 2022), which suggests alternative sources and/or multiple introduction events. The haplotype in Western Australia has yet to be determined.
Globally, 10 haplotypes of E. kuroshio have currently been documented, with 7 of those having been identified from Taiwan. Invasive populations of this species in California and Mexico constitute a single haplotype, H20, which is widespread in Taiwan (Rugman-Jones pers. communication).
Thirty-two haplotypes of the morphologically similar E. perbrevis (formerly E. fornicatus s. l.) have been documented worldwide. The introductions in Florida and Hawaii constitute a single haplotype, H8 (Stouthamer et al. 2017, Rugman-Jones et al. 2020), again hinting of a shared origin. In 2019, H8 was also collected in the Philippines, but the status of this species there (i.e., native or invasive) is not clear. The E. perbrevis haplotypes detected in nurseries in the Netherlands (reported as eradicated) constituted two additional haplotypes that are unknown elsewhere.
While many of these introductions occurred decades ago (e.g., Hawai`i, Florida, possibly Israel), a number of introductions were noticed around or after 2000 such as in California, South Africa, and a second species in Hawai`i. Bierman et al. (2022) note the constantly growing number of introduced locations.
Impact and Spread
California
The invasive shot hole borers first came to attention in late winter 2012, when a new insect/pathogen complex was discovered causing damage to avocado trees in residential neighborhoods and a commercial avocado grove in Los Angeles County, California. The insect was named polyphagous shot hole borer (PSHB). The disease is now called “Fusarium dieback”. Later studies determined that the beetle and its associated fungus had been in California since at least 2003, when it was found attacking black locust (Robinia pseudoacacia) (Eskalen et al. 2013).
Over a decade since the disease was discovered, the PHSB/pathogen complex has spread through significant parts of southern California. Meanwhile, an outbreak discovered in late 2013 in San Diego County was determined to be a second species of insect, the Kuroshio shot hole borer (KSHB) (California Forest Pest Council, 2015). Since it is a separate species and the initial detection site was separated by approximately 60 miles from the PSHB outbreak, authorities are confident that it resulted from a separate introduction.
As of spring 2022, the combined beetle/fungus complexes are throughout Orange, San Diego, Los Angeles, Riverside, San Bernardino and Ventura counties with outbreaks detected as far north as Santa Barbara /Santa Clarita (see the map here). Both the beetles and the plant pathogens they carry are expected to spread throughout much of California wherever their many host plants occur (UC IPM). The KSHB has “jumped” to more distant locations, including Santa Barbara, Ventura, San Luis Obispo, and Santa Clara counties. The two later detections apparently do not represent established populations.
Some California ecosystems are at particular risk because they are dominated by susceptible tree or shrub species. These vulnerable ecosystems are mixed evergreen forests, oak woodlands, foothill woodlands, & riparian habitats. In San Diego County alone, more than 58,000 acres of riparian woodlands are at risk (California Forest Pest Council).
The impact of disease appears to vary depending upon ecological factors. In the Tijuana River valley along the California-Mexico border, the KSHB initially caused considerable damage. In the “wet” forests close to perpetual streams in the Tijuana River delta, over the five years from 2015 to 2020, the beetle/fungus complex infested an estimate 350,000 willows and killed an estimated 123,000; willows near the main river channel had a fatality rate of 39%. This level of mortality supersedes that in other southern California deltas. More distant “dry forest” units had a combined fatality rate of only 9%. Dissection revealed that these trees had thicker bark which protected them by limiting the density of KSHB entry points. There might also be higher quantities of protective chemicals. Beginning in 2016, the trees in the Tijuana River estuary have regrown to almost pre-infestation dimensions. Boland (various studies) is not certain why these new, fast-growing trees have not been attacked by the KSHB which remains in the area. As expected, the damage to native willows led to proliferation of invasive plants, especially castor bean, salt cedar, and giant reed (Boland, 2018).
South Africa
PSHB has been present since at least 2012 (Stouthamer et al. 2017) in South Africa, although it was not identified until 2018. Within a decade it has spread to every province except Limpopo. This is the disease complex’ largest geographical outbreak of this beetle (Bierman et al. 2022).
Hawai`i
PSHB is attacking several endemic species in Hawai`i including one of the largest forest trees, Acacia koa, as well as Pipturus albidus and Planchonella sandwicensis. Numerous non-native species growing on the Islands are also attacked, including crops (Macadamia and Mangifera) and invasive species
(Ficus and Schinus molle) (Rugman-Jones et al. 2020).
South America
In 2023 PSHB was reported to be present in South America, specifically Buenos Aires, Argentina. An earlier report of the beetle being present in Brazil lacks specimens for verification (Ceriani-Nakamurakare, Johnson and Gomez, 2023). A large number of trees have succumbed to infestation, so it is suspected that the introduction occurred years ago. Several new host species have been identified, including tree species native to Argentina (Inga uraguensis, Schinus longifolia, and Solanum granuloso-leprosum). Two other native species, Inga vera and Tipuana tipu, were already known hosts. Eastern cottonwood (Populus deltoids), a tree widespread in eastern North America, has also been identified as a host (Ceriani-Nakamurakare, Johnson and Gomez, 2023).
The Argentine population of the beetle represents a haplotype most similar to specimens from Asia and greenhouses in Europe. Consequently, its origin differs from those of populations established in California, Israel, and South Africa (Ceriani-Nakamurakare, Johnson and Gomez, 2023). Ceriani-Nakamurakare and others (2023) also conclude that the beetle might pose a threat to urban and natural forests as well as to the forestry and fruit production industries.
Hosts
As many as 300 species have been named as hosts (Bierman et al. 2022); about a quarter of these are considered reproductive hosts – species that can support beetle reproduction and grow the fungi that cause Fusarium dieback (Eskalen et al. 2013). These species are in 33 plant families – a wide range that includes Aceracae, Fagaceae, and Salicaceae. However, those families also include species that apparently do not host the beetle or the fungus (Eskalen et al. 2016).
At least 65 tree species in southern California are reproductive hosts of one or both shot hole borers; globally, the number increases to 77 species (Bierman et al. 2022). Hosts identified in South Africa include Acer pseudoplatanus, Koelreuteria paniculata, Robinia pseudoacacia, Acalypha glabrata and Indigofera jucunda.
The preferred and most susceptible hosts are several species in the Acer, Parkinsonia, Platanus, Quercus, and Salix genera. Box elder (A. negundo) is so susceptible that it is considered a sentinel tree.
Hosts in California (see online list source here) as of Sept 2022 [Hosts Susceptible to ISHB-FD (may cause tree death) asterisk = native]:
- Acer buergerianum – Trident maple
- Acer macrophyllum – Big leaf maple*
- Acer negundo – Box elder*
- Parkinsonia aculeata – Palo verde
- Platanus racemosa – California sycamore*
- Platanus x hispanica – London plane
- Populus fremontii – Fremont cottonwood*
- Populus nigra – Black poplar*
- Populus trichocarpa – Black cottonwood*
- Quercus lobata – Valley oak*
- Quercus robur – English oak
- Ricinus communis – Castorbean
- Salix gooddingii – Black willow*
- Salix laevigata – Red willow*
- Salix lasiolepis – Arroyo willow*
51 species are listed as less susceptible to ISHB/FD (causes branch dieback), and/or the beetle commonly colonizes at the margin of canker diseases caused by other organisms.
Among those 51 species, three are native to California:
- Quercus agrifolia – Coast live oak*
- Quercus chrysolepis – Canyon live oak*
- Quercus engelmannii – Englemann oak*
Authorities have yet to explore the risk posed to other areas (e.g., the southeastern U.S.). Several of the high-risk hosts are common either in nursery trade or widespread in the Southeast – native or exotic:
- Acer palmatum – Japanese maple
- Ailanthus altissima – Tree of heaven
- Albizia julibrissin – Mimosa
- Camellia semiserrata – Camellia
- Casuarina equisetifolia – Australian pine tree
- Erythrina caffra – Coast coral tree
- Erythrina coralloides – Coral tree
- Erythrina falcata – Brazilian coral tree
- Fagus crenata – Japanese beech
- Gleditsia triacanthos – Honey locust
- Ilex cornuta – Chinese holly
- Jacaranda mimosifolia – Jacaranda
- Koelreuteria bipinnata – Chinese flame tree
- Liquidambar styriciflua – American sweet gum
- Magnolia grandiflora – Southern magnolia
- Magnolia virginiana – Sweet bay
- Persea americana – Avocado
- Wisteria floribunda – Japanese wisteria
As of 2023, nearly 600 species have been reported as hosts to PSHB worldwide (Ceriani-Nakamurakare, Johnson and Gomez, 2023).
Symptoms
Symptoms of PSHB attack and fungus infection differ among tree species. Click here for illustrations of the symptoms on various species.
Areas at Risk
Shannon Lynch and colleagues (Lynch et al. 2025) have developed a model to estimate vulnerability of urban areas based on phylogenetic structure (relationship between tree species), host abundance, and number of beetle generations/year (linked to temperature). They evaluated 170 California cities based on their tree inventories. According to this model, 87% of 832 urban forest grids from across California had a low probability of infestation. They found that infestation was possible only where temperatures favored an adequate number of beetle generations – at least five per year; this was true even in areas where competent host density was sufficient to support an infestation. The risk maps in Fig. 2 indicate higher risks in parts of southern California, drier regions of the San Francisco region, and portions of the Central Valley (Lynch et al. 2025). No one has yet applied this model to identify which Arizona and Nevada cities are most at risk.
In South Africa, conservative estimates were that 25% of urban trees would be lost (Bierman et al. 2022). By 2025, the complex has expanded from urban to wildland forests in eight of the country’s nine provinces (Lynch et al. 2025).
Management
Because the beetles spend most of their life inside trees, their life cycle leaves few opportunities for management intervention. While the females fly, they tend to bore galleries on their natal tree. Experts recommend focusing management on heavily infested “amplifier trees”. Dead limbs should be pruned. Dying trees and those with beetles infesting the main trunk should be removed. It is vital to dispose of infested wood properly; see advice posted here.
Experts also urge steps to prevent human assisted spread in dead wood, specifically green waste, firewood, and even large wood chips or mulch. These admonitions include references to buying firewood near where you will burn it and leaving any unused wood on site rather than moving it or bringing it home.
Scientists are exploring other possible strategies. Shannon Lynch (presentation to ISHB webinar April 2022; 2nd day) is studying whether endophytes might be used to kill the Fusarium fungi. Early results show some promise.
Richard Stouthamer (presentation to ISHB webinar April 2022; 2nd day) is exploring possible biocontrol agents. Of three he has evaluated, the most promising is Phymastichus sp., which is new to science. He is still trying to establish laboratory cultures so he can test its host specificity.
For more information on these pests, please visit:
• UC Invasive Shot Hole Borers (Polyphagous and Kuroshio) Information
• https://www2.ipm.ucanr.edu/Invasive-and-Exotic-Pests/Invasive-Shot-Hole-Borers/
——————————
*This profile benefited from significant input from Paul Rugman-Jones, Associate Project Scientist, Dept. of Entomology, University of California Riverside”
For more information on these pests, please visit:
- UC Invasive Shot Hole Borers (Polyphagous and Kuroshio) Information
- UC Riverside, Center for Invasive Species, PSHB Species Profile
- UC Riverside, Eskalen Lab research page
- https://peerj.com/articles/2141.pdf
——————————-
Sources
Bierman, A., F. Roets, and J.S. Terblanche. 2022. Population structure of the invasive ambrosia beetle, Euwallacea fornicatus, indicates multiple introductions into South Africa. Biol Invasions, 24:2301–2312. https://doi.org/10.1007/s10530-022-02801-x
Boland, J. Various reports and articles on the KSHB are available at: The Ecology and Management of the Kuroshio Shot Hole Borer in the Tijuana River Valley — Tijuana Estuary : TRNERR]
California Forest Pest Council. 2015. 2015 California Forest Pest Conditions. https://bofdata.fire.ca.gov/hot_topics_resources/2015_california_forest_pest_conditions_report.pdf
Ceriani-Nakamurakare, E., A.J. Johnson and D.F. Gomez. 2023. Uncharted Territories: First Report of Euwallacea fornicatus (Eichoff) in South America with new reproductive hosts records. Zootaxa, 5325 (2) 289-297. https://doi.org/10.11646/zootaxa.5325.2.10
Eskalen, A., Stouthamer, R., Lynch, S. C., Twizeyimana, M., Gonzalez, A., and Thibault, T. 2013. Host range of Fusarium dieback and its ambrosia beetle (Coleoptera: Scolytinae) vector in southern California. Plant Dis., 97:938-951.
Garcia-Avila, C.DJ., F.J. Trujillo-Arriaga, J.A. Lopez-Buenfil, R. Gonzalez-Gomez, D. Carrillo, L.F. Cruz, I. Ruiz-Galvan, A. Quezada-Salinas, and N. Acevedo-Reyes. 2016. First Report of Eucallacea nr. fornicatus (Coleoptera: Curculionidae) in Mexico. BioOne Complete, https://bioone.org/journals/florida-entomologist/volume-99/issue-3/024.099.0335/First-Report-of-Euwallacea-nr-fornicatus-Coleoptera–Curculionidae-in/10.1653/024.099.0335.full
Gomez, D.F., J. Skelton, M.S. Steininger, R. Stouthamer, P. Rugman-Jones, W. Sittichaya, R.J. Rabaglia, J. Hulcr. 2018. Species delineation within the Euwallacea fornicatus (Coleoptera: Curculionidae) complex revealed by morphometric and phylogenetic analyses. Insect Systematics and Diversity, 2:1-11. DOI 10.1093/isd/ixy018
Lynch, S.C., Reyes-Gonzalez, E., Bossard, E.L., Alarcon, K.S., Love, N.L.R., Hollander, A.D., Nobua-Behrmann, B.E., and Gilbert, G.S. A phylogenetic epidemiology approach to predicting the establishment of multi-host plant pests. Communications Biology, (8:117) 1-10.
Mitchell, A., and C. Maddox. 2010. Bark beetles (Coleoptera: Curculionidae: Scolytinae) of importance to the Australian macadamia industry: an integrative taxonomic approach to species diagnostics. Australian Journal of Entomology, 49:104_113. DOI 10.1111/j.1440-6055.2010.00746.x.
Normark, B. B., Jordal, B. H., and Farrell, B. D. 1999. Origin of a Haplodiploid Beetle Lineage. Proceedings: Biological Sciences, 266(1435), 2253–2259. https://www.jstor.org/stable/51655
Rabaglia, R.J., S.A. Dole, and A.I. Cognato. 2006. Review of American Xyleborina (Coleoptera: Curculionidae: Scolytinae) Occurring North of Mexico, with an Illustrated Key. Ann. Entomol. Soc. Am. 99: 1034–1056.
Rugman-Jones, P.F., M. Au, V. Ebrahimi, A. Eskalen, C.P.D.T. Gillett, D. Honsberger, D. Husein, M.G. Wright, F. Yousuf, and R. Stouthamer. 2020. One becomes two: second species of the Euwallacea fornicatus (Coleoptera: Curculionidae: Scolytinae) species complex is established on two Hawaiian Islands. PeerJ. 2020: 8:e9987
Smith, S.M., D.F. Gomez, R.A. Beaver, J. Hulcr, A.I. Cognato. 2019. Reassessment of the species in the Euwallacea fornicatus (Coleoptera: Curculionidae: Scolytinae) complex after the rediscovery of the “lost” type specimen. Insects 10:261 DOI 10.3390/insects10090261
Stouthamer, R., P. Rugman-Jones, P.Q. Thu, et al. 2017. Tracing the origin of a cryptic invader: phylogeography of the Euwallacea fornicatus (Coleoptera: Curculionidae: Scolytinae) species complex. Agric For Entomol 19:366-375. https://doi.org/10.1111/afe.12215
University of California. Pests and Diseases of southern California oak trees. https://ucanr.edu/sites/socaloakpests/Polyphagous_Shot_Hole_Borer/# accessed 24 June, 2013;
some presentations from September 2021 webinar posted at https://sustainability.uci.edu/pshb-uci/